Histopathology of Chironomus riparius (Diptera, Chironomidae) exposed to metal oxide nanoparticles
DOI:
https://doi.org/10.2298/ABS210515025SKeywords:
Chironomidae, histopathology, ecotoxicology, nanomaterials, biomarkersAbstract
Paper description:
- Chironomus riparius is a model organism in ecotoxicology. Histological analysis of its internal morphology could help identify biomarkers of the effects of sublethal concentrations of pollutants.
- 4th instar larvae of riparius were exposed to sublethal concentrations of TiO2, CeO2 and Fe3O4 nanoparticle and histological analysis was performed.
- Nano-Fe3O4 and nano-Fe3O4/nano-CeO2 exposure caused vacuolization of epithelial cells of midgut region I and Malpighian tubules, respectively; nano-TiO2 exposure altered fat body and midgut region II tissue architecture; nano-Fe3O4 shortened the intestinal brush border.
- The identified histopathological biomarkers of riparius larvae improve existing methodology in water quality assessment.
Abstract: As the production of metal-based nanomaterials increases, it is inevitable that nano-scale products and byproducts will enter the aquatic environment. In terms of global production, the most abundant nano-oxides are TiO2, CeO2 and Fe3O4 nanoparticles. Chironomus riparius is commonly used for ecotoxicological assessment and defining its histopathological biomarkers that showcase the toxic effect of tested nanoparticles should lead to a better understanding of the consequences of nanomaterial accumulation in aquatic ecosystems. In this study, a histological description of the digestive and excretory systems as well as the fat body structure of C. riparius larvae is provided. In addition, potential histological biomarkers of nano-oxide toxicity were determined based on the obtained histopathological alterations in organs. Vacuolization was observed in epithelial cells of midgut region I that were treated with nano-Fe3O4 as well as in Malpighian tubules treated with nano-Fe3O4 and nano-CeO2. Larvae exposed to nano-TiO2 showed alterations in the fat body and midgut region II tissue architecture. Additionally, shortening of the intestinal brush border was determined in groups exposed to nano-Fe3O4. These results reveal the high sensitivity of these organs, which can be used as biomarkers in histopathological assessment and therefore lead to further improvement of existing methodology in ecotoxicological studies.
Downloads
References
Stumm W. Water, an endangered ecosystem. Ambio. 1986;15(4):201-7.
International Organization for Standardization. ISO/TS 80004-1:2015. _Nanotechnologies — Vocabulary — Part 1: Core terms_ [Internet]. Geneva: ISO; 2015. [cited 2020 Jun 10]. Available from: https://www.iso.org/obp/ui/#iso:std:iso:ts:80004:-1:ed-2:v1:en
Wiechers JW, Musee N. Engineered inorganic nanoparticles and cosmetics: Facts, issues, knowledge gaps and challenges. J Biomed Nanotechnol. 2010; 6(5):408-31. https://doi.org/10.1166/jbn.2010.1143
Nel A, Xia T, Mädler L, Li N. Toxic potential of materials at the nanolevel. Science. 2006;311(5761):622-7. https://doi.org/10.1126/science.1114397
Thompson DT. Using gold nanoparticles for catalysis. Nano Today. 2007;2(4):40-3.
Theron J, Walker JA, Cloete TE. Nanotechnology and water treatment: applications and emerging opportunities. Crit Rev Microbiol. 2008;34(1):43-69.
Poynton HC, Robinson WE. Chapter 3.7 - Contaminants of Emerging Concern, With an Emphasis on Nanomaterials and Pharmaceuticals. In: Torok B, Dransfield T, editors. Green Chemistry: An Inclusive Approach. 1st ed. Elsevier; 2017. p. 291-315. https://doi.org/10.1016/b978-0-12-809270-5.00012-1
Mahaye N, Thwala M, Cowan DA, Musee N. Genotoxicity of metal based engineered nanoparticles in aquatic organisms: A review. Mutat Res Rev Mutat Res. 2017;773:134-60. https://doi.org/10.1016/j.mrrev.2017.05.004
Bondarenko O, Juganson K, Ivask A, Kasemets K, Mortimer M, Kahru A. Toxicity of Ag, CuO and ZnO nanoparticles to selected environmentally relevant test organisms and mammalian cells in vitro: A critical review. Arch Toxicol. 2013;87(7):1181-200. https://doi.org/10.1007/s00204-013-1079-4
Piccinno F, Gottschalk F, Seeger S, Nowack B. Industrial production quantities and uses of ten engineered nanomaterials in Europe and the world. J Nanoparticle Res. 2012;14(9):1109. https://doi.org/10.1007/s11051-012-1109-9
Weir A, Westerhoff P, Fabricius L, Hristovski K, Von Goetz N. Titanium dioxide nanoparticles in food and personal care products. Environ Sci Technol. 2012;46(4):2242-50. https://doi.org/10.1021/es204168d
Zdravkovic SD. Nanoparticles and potential aquatic ecosystems [dissertation]. Serbia: University of Nis; 2020. 346 p.
Jovanović B, Milosević D, Piperac MS, Savić A. In situ effects of titanium dioxide nanoparticles on community structure of freshwater benthic macroinvertebrates. Environ Pollut. 2016;213:278-82. https://doi.org/10.1016/j.envpol.2016.02.024
Arrêté du 17 avril 2019 portant suspension de la mise sur le marché des denrées contenant l’additif E 171 (dioxyde de titane - TiO2). J Off Rep Frnc. 2019;ECOC1911549A. French.
Dorier M, Béal D, Marie-Desvergne C, Dubosson M, Barreau F, Houdeau E, Herlin-Boime N, Carriere M. Continuous in vitro exposure of intestinal epithelial cells to E171 food additive causes oxidative stress, inducing oxidation of DNA bases but no endoplasmic reticulum stress. Nanotoxicology. 2017;11(6):751-61. https://doi.org/10.1080/17435390.2017.1349203
Dale JG, Cox SS, Vance ME, Marr LC, Hochella MF. Transformation of Cerium Oxide Nanoparticles from a Diesel Fuel Additive during Combustion in a Diesel Engine. Environ Sci Technol. 2017;51(4):1973-80. https://doi.org/10.1021/acs.est.6b03173
Zantye PB, Kumar A, Sikder AK. Chemical mechanical planarization for microelectronics applications. Mater. Sci. Eng. R; 2004;45(3-6):89-220. https://doi.org/10.1016/j.mser.2004.06.002
Zholobak NM, Shcherbakov AB, Bogorad-Kobelska AS, Ivanova OS, Baranchikov AY, Spivak NY, Ivanov VK. Panthenol-stabilized cerium dioxide nanoparticles for cosmeceutic formulations against ROS-induced and UV-induced damage. J Photochem Photobiol B Biol. 2014;130:102-8. https://doi.org/10.1016/j.jphotobiol.2013.10.015
Zhang F, Chan SW, Spanier JE, Apak E, Jin Q, Robinson RD, Herman IP. Cerium oxide nanoparticles: Size-selective formation and structure analysis. Appl Phys Lett. 2002;80(1):127-9. https://doi.org/10.1063/1.1430502
Goushbolagh NA, Farhood B, Astani A, Nikfarjam A, Kalantari M, Zare MH. Quantitative Cytotoxicity, Cellular Uptake and Radioprotection Effect of Cerium Oxide Nanoparticles in MRC-5 Normal Cells and MCF-7 Cancerous Cells. Bionanoscience. 2018;8(3):769-77. https://doi.org/10.1007/s12668-018-0538-z
Ganapathe LS, Mohamed MA, Yunus RM, Berhanuddin DD. Magnetite (Fe3O4) Nanoparticles in Biomedical Application: From Synthesis to Surface Functionalisation. Magnetochemistry 2020;6(4):68. https://doi.org/10.3390/magnetochemistry6040068
Liu G, Gao J, Ai H, Chen X. Applications and Potential Toxicity of Magnetic Iron Oxide Nanoparticles. Small. 2013;9(9-10):1533-45. https://doi.org/10.1002/smll.201201531
Giese B, Klaessig F, Park B, Kaegi R, Steinfeldt M, Wigger H, Von Gleich A, Gottschalk F. Risks, Release and Concentrations of Engineered Nanomaterial in the Environment. Sci Rep. 2018;8(1):1-18. https://doi.org/10.1038/s41598-018-19275-4
Ozmen M, Gungordu A, Geckil H. Ecotoxicity of Nanomaterials in Aquatic Environment. In: Thangadurai D, Sangeetha J, Prasad R, editors. Nanotechnology in the Life Sciences.1st ed. Cham: Springer; 2020. p. 351-77. https://doi.org/10.1007/978-3-030-31938-0_15
Uherek CB, Gouveia FBP. Biological Monitoring Using Macroinvertebrates as Bioindicators of Water Quality of Maroaga Stream in the Maroaga Cave System, Presidente Figueiredo, Amazon, Brazil. Int J Ecol. 2014;2014(1-2):1-7. https://doi.org/10.1155/2014/308149
Sanyal K, Deya A, Hazra N. Chironomids (Diptera) as Model Organisms: An Appraisal. In: Bandyopadhyay PK, editor. Proceedings of the National Conference on Challenges in Biodiversity and Resource Management [conference proceedings on the Internet]; 2013 Feb 26-27; Department of Zoology, University of Kalyani, District Nadia, West Bengal. Kalyani: University of Kalyani; 2014. [cited 2021 Jun 10]. p.188-193. Available from: https://www.researchgate.net/publication/280301706_Chironomids_Diptera_as_Model_Organisms_An_Appraisal
Stentiford GD, Longshaw M, Lyons BP, Jones G, Green M, Feist SW. Histopathological biomarkers in estuarine fish species for the assessment of biological effects of contaminants. Mar Environ Res. 2003;55(2):137-59. https://doi.org/10.1016/s0141-1136(02)00212-x
Ospina‐Pérez EM, Campeón‐Morales OI, Richardi VS, Rivera‐Páez FA. Histological description of immature Chironomus columbiensis (Diptera: Chironomidae): A potential contribution to environmental monitoring. Microsc Res Tech. 2019;82(8):1277-89. https://doi.org/10.1002/jemt.23278
Kumar V, Sharma N, Maitra SS. In vitro and in vivo toxicity assessment of nanoparticles. Int Nano Lett. 2017;7(4):243-56.
Zoroddu M, Medici S, Ledda A, Nurchi V, Lachowicz J, Peana M. Toxicity of Nanoparticles. Curr Med Chem. 2014;21(33):3837-53. https://doi.org/10.2174/0929867321666140601162314
Gurcan MN, Boucheron LE, Can A, Madabhushi A, Rajpoot NM, Yener B. Histopathological Image Analysis: A Review. IEEE Rev Biomed Eng. 2009;2:147-71. https://doi.org/10.1109/rbme.2009.2034865
Lavarías S, Arrighetti F, Siri A. Histopathological effects of cypermethrin and Bacillus thuringiensis var. israelensis on midgut of Chironomus calligraphus larvae (Diptera: Chironomidae). Pestic Biochem Physiol. 2017;139:9-16. https://doi.org/10.1016/j.pestbp.2017.04.002
Grueso-Gilaberth RN, Jaramillo-Timarán KS, Ospina-Pérez EM, Richardi VS, Ossa-López PA, Rivera-Páez FA. Histological Description and Histopathology in Polypedilum sp. (Diptera: Chironomidae): A Potential Biomarker for the Impact of Mining on Tributaries. Ann Entomol Soc Am. 2020;113(5):359-72. https://doi.org/10.1093/aesa/saaa008
Richardi VS, Vicentini M, Morais GS, Rebechi D, da Silva TA, Fávaro LF, Navarro-Silva MA. Effects of phenanthrene on different levels of biological organization in larvae of the sediment-dwelling invertebrate Chironomus sancticaroli (Diptera: Chironomidae). Environ Pollut. 2018;242:277-87. https://doi.org/10.1016/j.envpol.2018.06.091
OECD. OECD Guidelines for the Testing of Chemicals: Section 2, Test No. 235: Chironomus sp., Acute Immobilisation Test. p. 17. Paris: Organization for Economic Co-operation and Development; 2011. https://doi.org/10.1787/9789264122383-en
Richardi VS, Vicentini M, Rebechi D, Fávaro LF, Navarro-Silva MA. Morpho-histological characterization of immature of the bioindicator midge Chironomus sancticaroli Strixino and Strixino (Diptera, Chironomidae). Rev Bras Entomol. 2015;59(3):240-50. https://doi.org/10.1016/j.rbe.2015.07.003
Jovanović B, Bezirci G, Çağan AS, Coppens J, Levi EE, Oluz Z, Tuncel E, Duran H, Beklioğlu M. Food web effects of titanium dioxide nanoparticles in an outdoor freshwater mesocosm experiment. Nanotoxicology. 2016;10(7):902-12. https://doi.org/10.3109/17435390.2016.1140242
Savić‐Zdravković D, Milošević D, Uluer E, Duran H, Matić S, Stanić S, Vidmar J, Ščančar J, Dikic D, Jovanović B. A Multiparametric Approach to Cerium Oxide Nanoparticle Toxicity Assessment in Non‐Biting Midges. Environ Toxicol Chem. 2020; 39(1):131-140. https://doi.org/10.1002/etc.4605
OECD. OECD Guidelines for the Testing of Chemicals: Section 2, Test No. 218: Sediment-water Chironomid Toxicity Using Spiked Sediment. Paris: Organization for Economic Co-operation and Development; 2004. p. 21. https://doi.org/10.1787/9789264070264-en
Schneider CA, Rasband WS, Eliceiri KW. "NIH Image to ImageJ: 25 years of image analysis". Nature methods. 2012;9(7):671-5. https://doi.org/10.1038/nmeth.2089
Huang JH, Jing X, Douglas AE. The multi-tasking gut epithelium of insects. Insect Biochem Mol Biol. 2015;67:15-20. https://doi.org/10.1016/j.ibmb.2015.05.004
Costa MS, Cossolin JFS, Pereira MJB, Sant’ana AEG, Lima MD, Zanuncio JC, Serrão JE. Larvicidal and Cytotoxic Potential of Squamocin on the Midgut of Aedes aegypti (Diptera: Culicidae). Toxins (Basel). 2014;6:1169-76. https://doi.org/10.3390/toxins6041169
da Silva Costa M, de Paula SO, Martins GF, Zanuncio JC, Santana AEG, Serrão JE. Multiple Modes of Action of the Squamocin in the Midgut Cells of Aedes aegypti Larvae. PLoS One. 2016;11(8):e0160928. https://doi.org/10.1371/journal.pone.0160928
Holtof M, Lenaerts C, Cullen D, Vanden Broeck J. Extracellular nutrient digestion and absorption in the insect gut. Cell Tissue Res. 2019;377(3):397-414. https://doi.org/10.1007/s00441-019-03031-9
Chaika V, Pikula K, Vshivkova T, Zakharenko A, Reva G, Drozdov K, Vardavas AI, Stivaktakis PD, Nikolouzakis TK, Stratidakis AK, Kokkinakis MN, Kalogeraki A, Burykina T, Sarigiannis DA, Kholodov A, Golokhvast K. The toxic influence and biodegradation of carbon nanofibers in freshwater invertebrates of the families Gammaridae, Ephemerellidae, and Chironomidae. Toxicol Reports. 2020;7:947-54. https://doi.org/10.1016/j.toxrep.2020.07.011
Cintra-Socolowski P, Nocelli R, Roat T, Silva-Zacarin E, Malaspina O. Comparative physiology of Malpighian tubules: form and function. Open Acces Insect Physiol. 2016;6:13-23. https://doi.org/10.2147/oaip.s72060
Sorour J. Ultrastructural variations in Lethocerus niloticum (Insecta: Hemiptera) caused by pollution in Lake Mariut, Alexandria, Egypt. Ecotoxicol Environ Saf. 2001;48(3):268-74. https://doi.org/10.1006/eesa.2000.2003
de Almeida Rossi C, Roat TC, Tavares DA, Cintra-Socolowski P, Malaspina O. Effects of sublethal doses of imidacloprid in malpighian tubules of africanized Apis mellifera (Hymenoptera, Apidae). Microsc Res Tech. 2013;76(5):552-8. https://doi.org/10.1002/jemt.22199
Pintong A, Ampawong S, Komalamisra N, Sriwichai P, Popruk S, Ruangsittichai J. Insecticidal and Histopathological Effects of Ageratum conyzoides Weed Extracts against Dengue Vector, Aedes aegypti. Insects. 2020;11(4):224. https://doi.org/10.3390/insects11040224
Mittal S, Pandey AK. Cerium oxide nanoparticles induced toxicity in human lung cells: Role of ROS mediated DNA damage and apoptosis. Biomed Res Int. 2014;2014:891934. https://doi.org/10.1155/2014/891934
Nelliot A, Bond N, Hoshizaki DK. Fat-body remodeling in Drosophila melanogaster. Genesis. 2006;44(8):396-400. https://doi.org/10.1002/dvg.20229
Wu Y, Parthasarathy R, Bai H, Palli SR. Mechanisms of midgut remodeling: Juvenile hormone analog methoprene blocks midgut metamorphosis by modulating ecdysone action. Mech Dev. 2006;123(7):530-47. https://doi.org/10.1016/j.mod.2006.05.005
Aljedani DM. Comparing the histological structure of the fat body and malpighian tubules in different phases of honeybees, apis mellifera jemenatica (Hymenoptera: Apidae). J Entomol. 2018;15(3):114-24. https://doi.org/10.3923/je.2018.114.124
Even N, Devaud JM, Barron AB. General stress responses in the honey bee. Insects. 2012;3(4):1271-98. https://doi.org/10.3390/insects3041271
Wojciechowska M, Stepnowski P, Gołębiowski M. Cyfluthrin and Deltamethrin Induce Changes in the Fat Body Composition of Tenebrio molitor Larvae, Males and Females. Chem Biodivers. 2019;16(5):e1800515. https://doi.org/10.1002/cbdv.201800515
Fruttero LL, Leyria J, Moyetta NR, Ramos FO, Settembrini BP, Canavoso LE. The fat body of the hematophagous insect, Panstrongylus megistus (Hemiptera: Reduviidae): Histological features and participation of the β-chain of ATP synthase in the lipophorin-mediated lipid transfer. J Insect Sci. 2019;19(4). https://doi.org/10.1093/jisesa/iez078
Dalai S, Pakrashi S, Chandrasekaran N, Mukherjee A. Acute Toxicity of TiO2 Nanoparticles to Ceriodaphnia dubia under Visible Light and Dark Conditions in a Freshwater System. PLoS One. 2013;8(4):e62970. https://doi.org/10.1371/journal.pone.0062970
Castagnola A, Jurat-Fuentes JL. Intestinal regeneration as an insect resistance mechanism to entomopathogenic bacteria. Curr Opin Insect Sci. 2016;15:104-10. https://doi.org/10.1016/j.cois.2016.04.008
Li-Byarlay H, Pittendrigh BR, Murdock LL. Plant Defense Inhibitors Affect the Structures of Midgut Cells in Drosophila melanogaster and Callosobruchus maculatus. Int J Insect Sci. 2016;8(8):71-9. https://doi.org/10.4137/ijis.s28595
Friederich E, Louvard D. Microvilli. In: Ganten D, Ruckpaul K, editors. Encyclopedic Reference of Genomics and Proteomics in Molecular Medicine. 1st ed. Berlin: Springer; 2006. p. 1116-21. https://doi.org/10.1007/3-540-29623-9_4120
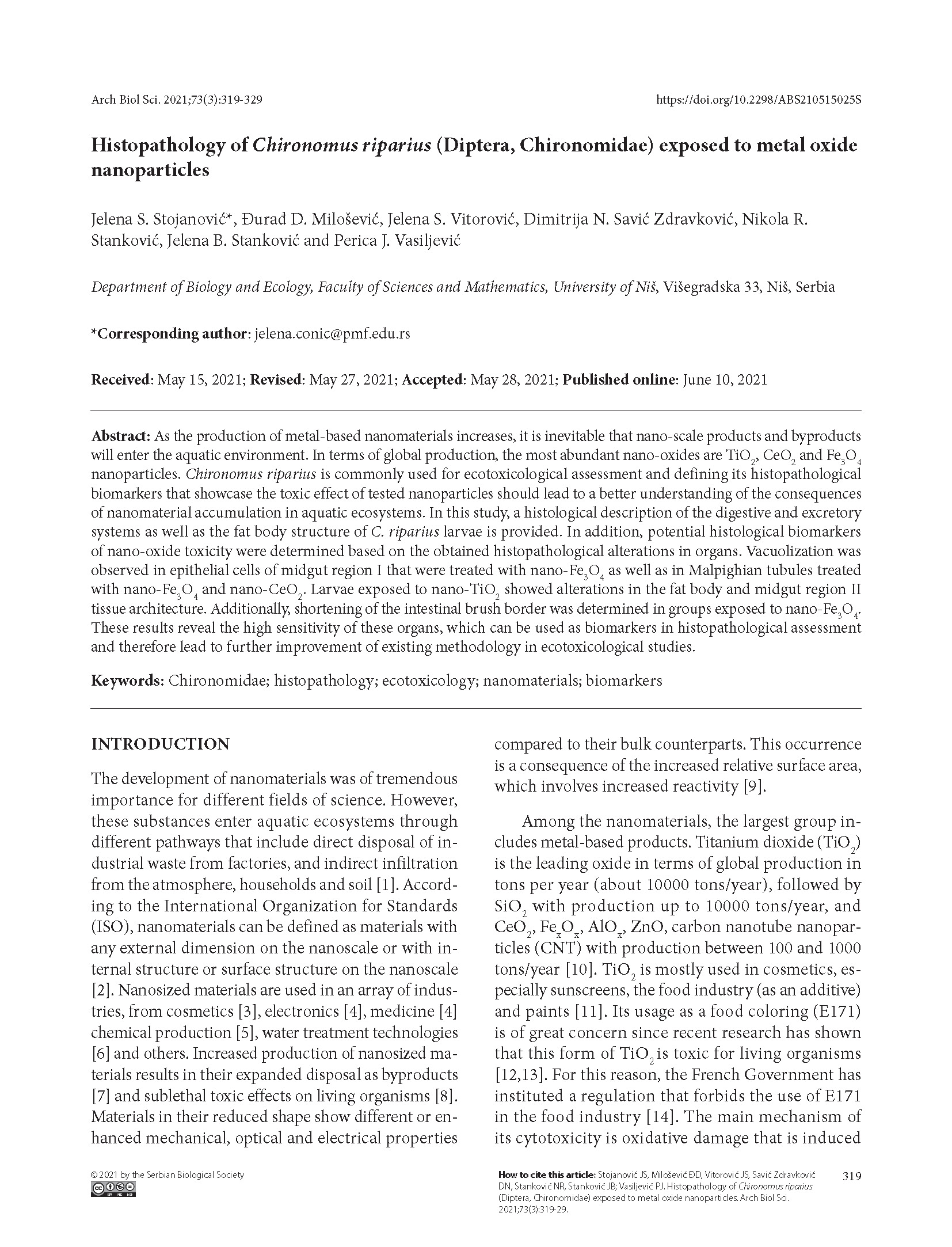
Downloads
Published
How to Cite
Issue
Section
License
Copyright (c) 2021 Archives of Biological Sciences

This work is licensed under a Creative Commons Attribution-NonCommercial-NoDerivatives 4.0 International License.
Authors grant the journal right of first publication with the work simultaneously licensed under a Creative Commons Attribution 4.0 International License that allows others to share the work with an acknowledgment of the work’s authorship and initial publication in this journal.